Understanding Vertical Ventilation Shafts Design Principles for Basement Air Exchange Systems
Understanding Vertical Ventilation Shafts Design Principles for Basement Air Exchange Systems - Stack Effect Principles for Natural Air Movement in Basement Systems
The stack effect, sometimes called the chimney effect, naturally moves air within buildings because of pressure, temperature, and density differences between indoors and outdoors. Vertical shafts can boost this natural ventilation, promoting upward air movement and bringing in fresh air to improve quality. Efficient designs using the stack effect need to consider factors like building height, where openings are placed, and the local climate. Placing openings at both the top and bottom of a space helps air flow, with warm air going out the top and cool air coming in the bottom. Things like chimneys, vents, atriums, and windows that open can be used to design these systems for the best air movement. Software for modeling and simulation has gotten better at letting us measure how well these natural ventilation methods work, which helps in designing and using the stack effect. Good natural ventilation is not only more comfortable but also healthier by removing pollutants. Sometimes though designers might rely too heavily on traditional rules of thumb which may not always translate well into unique building designs, potentially leading to suboptimal ventilation strategies. Besides the stack effect, the Venturi effect and cross ventilation can also be used to help move air inside a building. The size of the openings is important as it directly affects how much air is exchanged, with larger openings generally being more efficient. Understanding the stack effect is vital for those designing heating and cooling systems to make sure ventilation works at its best in building projects.
The stack effect is essentially a naturally occurring phenomenon that leverages differences in air density due to temperature gradients to induce airflow within a building. This can be quite pronounced in basements. It's like nature's own ventilation system, but how well does it really work in practice? One can observe that when the inside of a building is warmer than the outside, the less dense, warmer indoor air tends to rise and escape through openings near the top. This creates a lower pressure area inside, pulling cooler, denser outside air in through lower openings. It's fascinating how this simple temperature difference can drive such movement. It would be interesting to find a solid set of studies quantifying this in detail. Designing ventilation systems that capitalize on this effect seems straightforward enough, by strategically placing inlets and outlets. However, optimizing these systems for real-world performance requires a deeper understanding of several variables, which could use more study. The height of the ventilation path, or in simpler words the vertical distance between air inlets and outlets, will certainly play a role and is something that engineers have to consider. Also of note is that the local climate conditions exert a considerable influence, potentially leading to unpredictable performance outcomes. A proper analysis and simulation tool seems like a must. In many cases it seems the size of the openings is critical. Intuitively, bigger openings should facilitate more air exchange, but at what point does this become counterproductive or create other issues? Further research is needed on optimal sizing. And let's not forget the Venturi effect, which is a different, yet related, phenomenon that could be utilized alongside the stack effect to further enhance air movement. How these two principles interact in complex building designs is an intriguing area of study, but it is still hard to grasp. Overall, while the principles behind the stack effect are well-documented in a way, their application in modern building design, especially in basements, still poses interesting challenges that warrant further investigation. It seems obvious that there are lots of opportunities to fine tune the use of these effects, but there are a lot of claims out there that do not seem substantiated by strong evidence.
Understanding Vertical Ventilation Shafts Design Principles for Basement Air Exchange Systems - Air Exchange Rate Calculations for Underground Ventilation Design
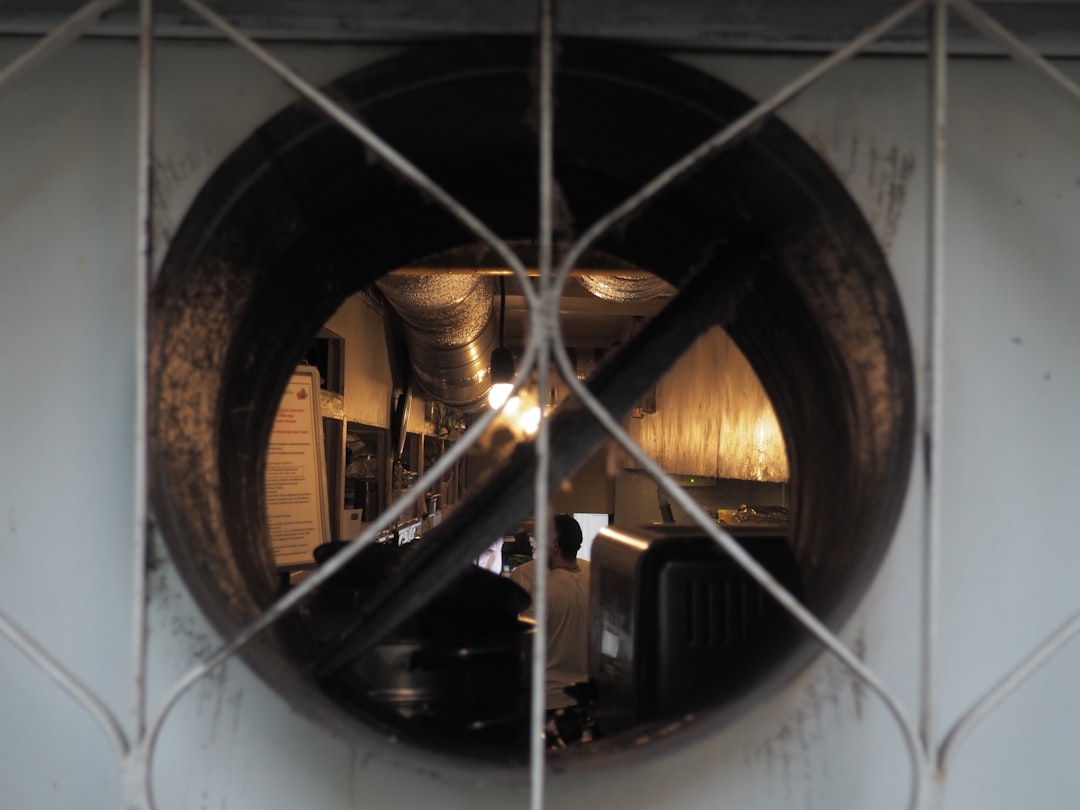
Calculating air exchange rates is crucial when designing ventilation for underground spaces. This directly affects the quality of the air inside. The air exchange rate, or ACH, tells us how often the air in a room is completely replaced. It's a key measure, especially in places like basements where fresh air might be hard to come by. When designing ventilation shafts, it is good to think about using both natural airflow and mechanical systems to move air around properly. The way these shafts are positioned and how big they are really matters. There are also guidelines that say how much fresh air needs to come in from outside, depending on the building. But it is sometimes tough to follow these rules exactly in every design. It is always a good idea to carefully work out these air exchange calculations to make sure people in these enclosed spaces are comfortable and healthy, and this does not always happen. Sometimes the calculations are not that precise. One method for calculating is pressurization testing which can find leaks that let air escape, messing up the calculations, but it is not always that precise. Another method is putting exhausts near things that make the air dirty, but sometimes the placement is not optimal. In any case, this is what is new with air exchange rate calculations.
Air exchange rates are critical when designing underground ventilation systems, typically measured in air changes per hour, or ACH. This metric indicates how often the entire air volume within a space is replaced in one hour. It would be very helpful to see the equation in practice with some real world scenarios. It is commonly stated that a well-designed system should achieve an ACH of 6 or higher for adequate pollutant removal and thermal comfort. Yet, achieving this in confined underground spaces with potential airflow blockages seems like a significant challenge. I wonder how often these targets are actually met in practice. The height of vertical shafts is another important consideration. It is claimed that a 10% increase in shaft height can boost airflow by up to 30% due to an enhanced stack effect. This seems substantial; however, is there any data showing the boundary conditions of this effect? Adjustments for air density variations caused by temperature and humidity changes are also necessary, with a 1% increase in humidity potentially reducing air density by about 0.2%. How significant is this in real-world applications? It seems like many variables at play that all seem to have a relatively minor effect. But is it really minor when you consider them all together? The shape and orientation of openings are apparently critical factors, with square openings often providing better airflow than circular ones due to a greater effective flow area. There are likely several studies that support this. Incorporating mechanical systems, such as small fans, alongside natural ventilation driven by the stack effect, can potentially enhance air exchange rates without a huge energy cost. It would be interesting to explore the optimal balance between natural and mechanical ventilation. Computational fluid dynamics (CFD) simulations have become popular for predicting airflow patterns. How accurate are these models, and do they adequately account for real-world variables like turbulence and non-uniform airflow? I suspect that many underground ventilation systems in the real world fall short of their designed ACH rates. This suggests a potential gap between theoretical models and actual performance. It seems like much of the current design approach relies heavily on empirical data and established formulas. This makes one question whether we need more comprehensive, context-specific research to create more effective ventilation designs. This area really needs a lot more study and perhaps a rethink of how things are being done.
Understanding Vertical Ventilation Shafts Design Principles for Basement Air Exchange Systems - Material Selection and Construction Methods for Vertical Shafts
Material selection and construction methods for vertical shafts are crucial elements in the design of effective basement ventilation systems. The choice of materials must prioritize both durability and thermal performance to optimize airflow and resist environmental challenges, such as moisture and soil instability. It is sometimes said that concrete is a great choice, but this may not always be the case depending on the soil conditions. Construction techniques can vary widely, from traditional excavation to modern mechanized methods that address challenges posed by groundwater and heterogeneous soils. Some modern techniques though still need some time to mature. It is essential that designers carefully consider these factors to ensure that the shafts not only serve their primary function of facilitating air exchange but also maintain structural integrity over time. Often times structural integrity is the main focus, and sometimes thermal performance takes a backseat. An integrated approach that balances material properties with innovative construction practices can significantly enhance the overall performance of vertical shafts in promoting healthy indoor environments. It is good to see that there are a lot of innovative construction practices out there that take into account a variety of concerns.
When picking materials for building vertical shafts, it seems obvious that durability should be a top priority. Things like reinforced concrete are often touted for their ability to handle pressure and resist corrosion, especially in the damp, challenging conditions found underground. But how well do these materials truly hold up over decades? There are claims about their longevity, but I wonder about the real-world data, especially considering issues like water infiltration and unexpected chemical reactions. It seems like some materials might degrade faster than predicted. Then there is the thermal conductivity of the chosen materials, which surely affects temperature gradients within the shaft. Some materials are better than others at preventing heat transfer, and it seems like this would directly impact the efficiency of the ventilation system. But how much does it really matter in practice? One would need to dig deeper into the research to find quantifiable impacts on air exchange rates. Noise control is another interesting aspect. In busy urban areas, it seems vital to use sound-absorbing materials to minimize noise transmission from these shafts. There are probably standards and regulations around this, but how effectively are they enforced, and do they make a noticeable difference to residents? In places prone to fires, the choice of non-combustible materials seems non-negotiable. Beyond just safety, these materials reportedly help prevent the spread of smoke, a claim that appears well-supported by fire safety studies. One would hope that the selection of such materials is standard practice, but are there instances where corners are cut? Moving to construction techniques, methods like slipforming are praised for reducing joints and weak points. Fewer joints should mean fewer places for potential failure, in theory improving the shaft's longevity. Yet, how does this method compare to others in terms of cost-effectiveness and long-term performance? It seems like it is generally superior, but an engineer has to wonder. Engineers also need to account for loading conditions and pressure differentials, which can affect the shaft's stability and ventilation efficiency. The interaction between axial and lateral loads is complex, particularly in earthquake-prone areas. Are current design models fully capturing these interactions, or are there gaps that could lead to structural issues down the line? The stack effect is often mentioned in relation to pressure differentials, but how often are these optimal conditions met in real-world designs? Suboptimal designs leading to poor ventilation performance seem like a common problem that is not being addressed. Finally, the practical aspects of maintenance access and integration with other building systems cannot be overlooked. It is almost comical how frequently access points for maintenance are underestimated in their importance. Without proper access, how can these shafts be effectively inspected and maintained? It is claimed that ease of maintenance can greatly extend the lifespan of air exchange systems. Integration with other building systems, such as plumbing and electrical, also seems crucial for avoiding operational inefficiencies and hazards. How often do these integrations go wrong, and what are the consequences? It seems like a simple thing that should be kept in mind, but humans make mistakes. These are all important considerations, but the real question is how well are they being addressed in practice? There is a lot of room for research and improvement in this field, which needs to take a fresh look at some of the claims out there.
Understanding Vertical Ventilation Shafts Design Principles for Basement Air Exchange Systems - Temperature Differential Management Through Shaft Placement
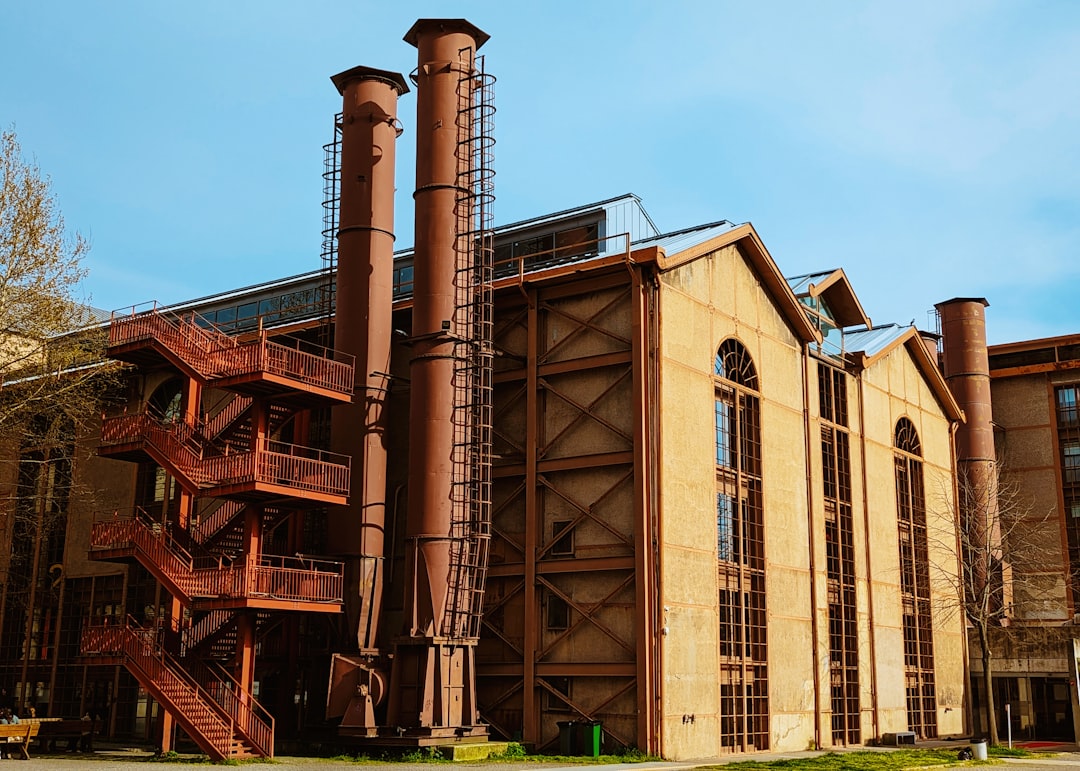
Temperature differential management through strategic placement of vertical ventilation shafts is critical in optimizing basement air exchange. These shafts help move warm air upwards, enhancing the natural stack effect and improving smoke evacuation during fires. Temperature variations affect smoke and gas flow dynamics. The placement and design angles of these shafts significantly impact their performance in both normal and emergency situations. It all seems to work well in principle, but there are lots of variables that are not being considered. Relying on set design principles without considering site-specific variables can lead to inefficient systems that fail to meet real-world needs. Integrating ongoing research and adaptive design is essential for effective temperature management and overall safety in basement air exchange systems. There is a real need for rigorous testing under a variety of conditions to validate these designs. It is concerning that many designs do not seem to undergo such testing. A more nuanced approach is needed.
Temperature differences within ventilation shafts significantly influence how well they work, especially regarding the stack effect. It is often mentioned that a mere 2°C differential can initiate airflow, but how consistently does this occur in real-world scenarios? Variations throughout the day can reportedly lead to a 30% fluctuation in ventilation performance, suggesting that reliance on such a narrow temperature range might be overly optimistic. Also of note is that the height of these shafts plays a critical role, with a commonly cited minimum of 3 meters needed to achieve any meaningful stack effect. This raises questions about the practicality of shorter shafts in certain building designs. Are they merely vestigial structures in such cases? The interaction between temperature gradients and shaft height seems like a complex interplay. Furthermore, the shape of the shaft's cross-section is often discussed, with claims that square or rectangular designs might outperform circular ones. The aerodynamic principles behind these assertions are intriguing, but one wonders about the empirical evidence supporting such claims, especially across various building types and climates. There is also the issue of pressure variations, which are apparently influenced by external wind conditions. It is stated that a 1 m/s increase in wind speed can significantly boost air exchange rates, yet how reliable are these figures when considering the unpredictable nature of wind in urban environments? The formation of vortices at shaft openings is a particularly interesting point. These swirling air patterns can apparently create negative pressure zones, potentially disrupting the intended airflow. How often does this phenomenon occur, and to what extent does it compromise ventilation efficiency? It seems like a critical factor that is difficult to predict. Integrating these shafts with existing HVAC systems also presents its own set of challenges. There is a potential for poorly aligned systems to negate the benefits of natural airflow, possibly leading to stagnant air pockets. How frequently do such misalignments occur in practice, and what are the implications for indoor air quality? This seems like a significant oversight in many designs. Moreover, while concrete is commonly favored for shaft construction, emerging data suggests that steel might offer superior thermal performance in certain cases. The specifics of these scenarios are not often clear, particularly regarding how different climates and humidity levels come into play. Lastly, airflow within shafts is rarely uniform, often exhibiting complex patterns like swirling and recirculation. Computational fluid dynamics (CFD) modeling is highlighted as a tool to predict these behaviors, but how accurately can these models reflect real-world conditions? This makes one skeptical about their practical utility in everyday design scenarios. In conclusion, while managing temperature differentials through strategic shaft placement is theoretically sound, the practical implementation appears fraught with complexities and potential pitfalls. It is a field ripe for further research and critical analysis. This field has a long way to go.
Understanding Vertical Ventilation Shafts Design Principles for Basement Air Exchange Systems - Cross Ventilation Integration with Vertical Shaft Systems
Cross ventilation integration with vertical shaft systems offers a potentially effective way to improve air exchange in basements. This approach uses strategically placed openings on opposite sides of a building to bring in fresh air and remove stale air. This works by utilizing natural airflow from differences in temperature and pressure. However, how well these systems work depends a lot on local weather, the right size of openings, and the heat performance of materials used for the shafts. It is also crucial to consider how these vertical shafts work with existing heating and cooling systems. Problems can arise if they are not properly aligned, leading to inefficiencies that cancel out any benefits. While cross ventilation can potentially improve indoor air quality and energy efficiency, the complexity and varying performance in different situations highlight the need for more research and better design standards.
Integrating cross ventilation with vertical shaft systems presents a curious blend of opportunities and challenges. The idea that dynamic pressure variations, influenced by wind and surrounding structures, can enhance cross ventilation is fascinating. It seems intuitive that careful site assessments would be crucial to make the most of these natural forces. But how often are these assessments truly comprehensive? One might suspect that practical constraints often lead to compromises. The geometric shape of these shafts also seems to play a vital role. It is claimed that rectangular shafts can outperform circular ones, which is intriguing. The thought that a larger surface area could facilitate better initial airspeed makes sense, but one wonders about the real-world data supporting this. Are there comparative studies across various building types and climates? It seems like an area ripe for deeper investigation.
Temperature gradients are also a critical factor, with even a tiny 1-2°C difference supposedly initiating air movement. This is a remarkably small margin, and it makes one question how realistic it is to maintain such conditions consistently. It is easy to imagine scenarios where this delicate balance is disrupted, leading to suboptimal performance. As for shaft height, the conventional wisdom suggests a minimum of 3 meters for effectiveness. This seems reasonable, given the physics of the stack effect. However, it begs the question: what about designs with shorter shafts? Are they simply ineffective, or is there a way to optimize their performance? The impact of humidity on air density is another intriguing point. It is said that a mere 5% increase in humidity can reduce ventilation efficiency. This highlights the importance of considering local climate conditions in the design process. Yet, how often is this level of detail truly incorporated into real-world projects?
Wind influence is clearly a major factor, with claims that a 1 m/s increase in wind speed can significantly boost ventilation rates. This is impressive, but the unpredictable nature of wind, especially in urban environments, adds a layer of complexity. It seems like a challenge to design systems that can reliably harness such variable forces. The formation of vortices at shaft openings is also a concern, potentially creating negative pressure zones that disrupt airflow. This phenomenon seems like a critical factor that could easily be overlooked, and it would be interesting to see more research on its impact. The integration of these shafts with existing HVAC systems is another area where things can get tricky. Misalignment can apparently lead to stagnant air pockets and reduced efficiency. This seems like a significant oversight in many designs, and it makes one wonder how often these systems are truly working in harmony.
Computational fluid dynamics (CFD) modeling is often touted as a powerful tool for predicting airflow. However, its real-world accuracy is sometimes questioned, particularly its ability to account for complex factors like turbulence. It seems like a useful tool, but perhaps not a silver bullet. Finally, the choice of materials, such as reinforced concrete, is often discussed in terms of longevity. But how well do these materials truly hold up under real-world conditions? Factors like moisture and chemical reactions could potentially compromise their integrity over time. It is concerning that some materials might degrade faster than predicted, suggesting a need for more rigorous, long-term assessments. There is a lot of talk about innovative designs and materials, but are we truly seeing these advancements translate into better, more resilient ventilation systems? Or are we still grappling with fundamental challenges that have yet to be adequately addressed? The field appears to be filled with potential, yet there is a lingering sense that we have only scratched the surface of what is truly possible.
Understanding Vertical Ventilation Shafts Design Principles for Basement Air Exchange Systems - Safety Standards and Building Code Requirements for Basement Ventilation
Basement ventilation is governed by a set of safety standards and building codes aimed at ensuring good air quality and the well-being of those inside. Various building codes, including the International Mechanical Code and International Residential Code, provide rules for both natural and mechanical ventilation, specifying the amount of fresh air required per person. Following these codes is vital to prevent problems like mold and bad air quality, which can happen with poor ventilation. Ventilation system designs must follow these rules and consider the specific environmental conditions of each location to work best. Dealing with these codes and standards is a big challenge for designers to make sure basement air exchange systems are both effective and safe. It is sometimes said that following these codes is straightforward, but each site presents unique challenges. The specific requirements outlined in sections like R303, M1505, and G2407 of the IRC often seem clear in theory but can be difficult to implement perfectly in practice. The codes often mandate a range of 5 to 10 cubic feet per minute of fresh air per person, but achieving this consistently across different basement layouts and uses is easier said than done. Mechanical ventilation systems, which actively move air using fans, are frequently recommended. Yet, their installation and maintenance can be more complex than anticipated. Natural ventilation, relying on passive airflow through windows and vents, is simpler but its effectiveness varies greatly with external conditions, making it sometimes unreliable. Guidelines from organizations that set standards offer a framework for maintaining air quality, but these are not always easy to apply uniformly. Ventilation rates are supposed to be adjusted based on how many people are using the space and what they are doing, a requirement that is hard to keep track of in real-world settings. Moreover, the design must prevent the buildup of pollutants from outside by carefully positioning air intakes and exhausts, a task that is not always executed flawlessly in practice. The reality is that while these standards aim to create safe and healthy environments, the actual implementation often reveals gaps between theory and practice, underscoring the need for ongoing research and adaptive design approaches in the field of basement ventilation. There are instances where it appears that shortcuts are taken, leading to suboptimal outcomes that do not fully meet safety or health standards.
Building codes for basement ventilation are a patchwork, varying significantly from place to place. It seems this is largely due to local climate and geological differences. This makes things tricky for engineers who might be working on projects across different regions, potentially leading to inconsistent safety and performance standards. It is a bit concerning that there is not a more unified approach, especially when dealing with something as crucial as air quality. Pressure differences between the basement and the outside are also a big deal. It is stated that exceeding a pressure delta of 7.5 Pa can lead to air infiltration issues, which would surely complicate the effectiveness of ventilation. This seems like a precise threshold, but how often is it actually monitored in practice? It is hard to grasp that this is being given adequate attention in the field. Properly designed vertical shafts are not just about air exchange. They are critical for smoke and heat evacuation during fires. It is interesting that codes require smoke ventilation in certain buildings, highlighting the dual role of these systems. But this makes one wonder how well these systems are actually tested under real fire conditions. Do they truly perform as intended, or are there gaps between theory and practice?
Moisture management is another key aspect. High humidity can apparently reduce air density by about 1% for every 10% increase in humidity, impacting the stack effect. It seems engineers need to consider moisture barriers, but how effective are these in the long run? It would be interesting to see data on how these barriers perform over time in various climates. Airflow within shafts is rarely uniform, often showing complex patterns like swirling. It is mentioned that Computational Fluid Dynamics (CFD) is used to model this, but it seems these models often fall short in capturing real-world complexities. This makes one skeptical about relying too heavily on these simulations for design decisions. The recommended air change rate in basements is at least 6 ACH. However, it appears that achieving this in underground spaces is challenging. It would be helpful to see more data on how often this target is actually met in practice, and what factors contribute to success or failure. This seems like a critical area that needs more attention.
Materials like reinforced concrete are commonly used for their strength, but there are concerns about their long-term performance. Studies suggest they can fail early due to moisture and chemical reactions. It would be valuable to see more research on alternative materials that might offer better longevity and performance in these environments. This is something that is in dire need of more research. Integrating cross-ventilation with existing HVAC systems is vital but challenging. Misalignments can lead to stagnant air, undermining the system's performance. It seems like a complex puzzle, and it would be interesting to know how often these integrations truly achieve optimal results. There are probably more failures than successes on this front. Given the variability of conditions, adaptive design approaches seem necessary. However, it is noted that this is often overlooked, leading to systems that do not meet real-world needs. This suggests a gap between current design practices and what is actually required for effective ventilation. It seems obvious that the field could benefit from more dynamic design strategies.
Finally, many building updates might not undergo rigorous testing against current codes. This allows outdated practices to persist, leading to suboptimal designs that impact occupant health. It is a bit alarming that this is not being addressed more systematically. There is clearly a need for more stringent enforcement and testing of these systems. There is a lot of discussion about standards and guidelines, but how well are they really being followed? It is easy to imagine that corners are being cut, particularly when there are cost and time pressures. This whole area seems ripe for further investigation and improvement, and it is crucial that we do not lose sight of the ultimate goal: ensuring safe and healthy indoor environments.
More Posts from archparse.com: